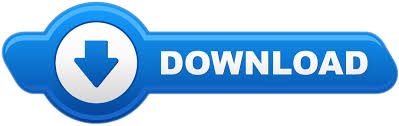
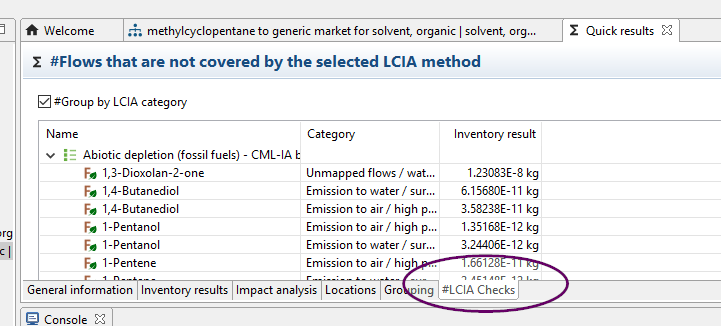

Depending upon the functional unit, the other products and/or services are modeled through system boundary expansion. In the TVR process, there are many co-products or services provided: disposal of used tires, production of light fuel feedstock, production of heavy fuel feedstock, production of carbon black and production of steel. In addition, Monte Carlo simulations were used to understand the statistical reliability of the results with respect to the data quality, and results were only reported if they were consistent across 95% or more of the comparative simulations.
OPENLCA CML BIOGENIC CARBON ISO
This comparative LCA study, which underwent an ISO compliant panel critical review, included the use of midpoint (TRACI) and damage (ReCiPe Endpoint) categories. Life cycle assessment (LCA) was used to examine the life cycle impacts of the TVR process relative to conventional tire disposal in an incinerator or landfill, as well as to quantify the production of several useful co-products generated in the TVR process.Īttributional LCA was used to analyze the TVR system from multiple vantage points to understand the TVR system from an operating perspective, as a disposal service for waste tires and as a production method for fuel feedstocks. Green Carbon has developed a thermal vacuum recovery (TVR) system to recycle on-road and off-road tires and tracks and process them into high carbon steel, carbon black, two grades of fuel feedstocks and a hydrocarbon-rich gas (process gas), which is used to heat the process. We present a decision-tree framework outlining proposed steps needed to enhance HH CF modeling incorporating new techniques to rapidly estimate chemical exposure and effects.ġ. This requires incorporating advances in rapid near-field exposure modeling and source characterization.

Using computer modeled toxicities, however, may provide the most practical solution for a priori HH effect factor predictions, which could be used with modeled exposure factors to derive CFs.Īs exposure is also a key component of HH CFs, we also discuss the inclusion of use-phase near-field sources in LCIA. The bioactivities derived from ToxCast have been combined with pharmacokinetic and exposure modeling to rank and prioritize chemicals for risk assessment, and this type of analysis may lend itself to LCIA techniques, which are comparative in nature.
OPENLCA CML BIOGENIC CARBON SOFTWARE
An example of this is the Toxicity Estimation Software Tool (TEST), also developed at the EPA, which allows for rapid chemical toxicity estimation based on chemical structure properties. Computational models can be used to estimate chemical toxicity, for example using quantitative structure activity relationships (QSARs). In recent years, the EPA developed the Toxicity Forecaster (ToxCast) program, which uses high-throughput in vitro assay methods to estimate chemical bioactivity for thousands of chemicals without the use of animals. We explore incorporating advances near-field exposure modeling and chemical toxicity estimation techniques into HH impact assessment. This poses a challenge for incorporating HH impacts into LCIA, especially during the product design phase when focusing on newly developed chemicals or chemical alternatives. This method is thus limited to chemicals without near-field releases and to those with empirically derived toxicity factors and is not applicable to chemicals with little or no effect data available. In the widely used USEtox LCIA framework, exposure is characterized via modeled intake fractions which are focused on far-field chemical releases, and toxicity is characterized via effect factors, which have been derived from experimental toxicity studies. Within Life Cycle Impact Assessment (LCIA), characterization factors (CFs) for chemically- mediated human health (HH) impacts are a combination of exposure and toxicity.
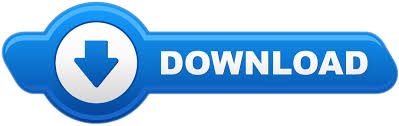